A Guide to the Interfaces of Optical Transceiver Modules
In today's optical communications market, there are a variety of transceiver modules with various types of interfaces. Because different types of cables/connectors/adapters are required for different interfaces, we need to pay more attention when selecting the relevant assemblies. This article will give you a detailed introduction to the mainstream transceiver module interfaces on the market, so that everyone has a clearer understanding of the transceiver modules.
First of all, we use the following table to list all transceiver modules' interfaces.
Form Factor |
Transmission Mode |
Interface |
Example |
QSFP-DD |
Parallel |
MPO |
200G QSFP-DD SR8/PSM8 |
QSFP-DD |
Multiplexing |
Dual CS |
200G QSFP-DD CWDM8 |
QSFP28 |
Parallel |
MPO |
100G QSFP28 SR4/PSM4 |
QSFP28 |
Multiplexing |
Duplex LC |
100G QSFP-DD LR4/CLR4/CWDM4/ER4 |
QSFP+ |
Parallel |
MPO |
40G QSFP+ SR4/PSM4 |
QSFP+ |
Multiplexing |
Duplex LC |
40G QSFP+ LR4 |
SFP28 |
Dual Fiber |
Duplex LC |
25G SFP28 SR/LR |
SFP28 |
Single Fiber Bidirectional |
Simplex LC |
25G SFP28 BiDi |
SFP+ |
Dual Fiber |
Duplex LC |
SFP+ 10GBASE-SR/LR |
SFP+ |
Single Fiber Bidirectional |
Simplex LC/SC |
SFP+ BiDi |
SFP+ |
2-channel Bidirectional |
Dual LC |
SFP+ 2-channel BiDi |
SFP+ |
Electrical Copper Cable |
RJ-45 |
SFP+ 10GBASE-T |
SFP |
Dual Fiber |
Duplex LC |
SFP 1000BASE-SX/LX |
SFP |
Single Fiber Bidirectional |
Simplex LC/SC |
SFP BiDi |
SFP |
2-channel Bidirectional |
Dual LC |
SFP 2-channel BiDi (CSFP) |
SFP |
Electrical Copper Cable |
RJ-45 |
SFP 1000BASE-T |
CXP |
Parallel |
MPO |
120G CXP SR10 |
CFP |
Parallel |
MPO |
100G CFP SR10 |
CFP |
Multiplexing |
Duplex LC |
100G CFP LR4/ER4 |
CFP2 |
Parallel |
MPO |
100G CFP SR10 |
CFP2 |
Multiplexing |
Duplex LC |
100G CFP2 LR4/ER4 |
CFP4 |
Parallel |
MPO |
100G CFP4 SR4 |
CFP4 |
Multiplexing |
Duplex LC |
100G CFP4 LR4/ER4 |
As the table shows, although there are more than a dozen types of transceiver modules, there are only a few types of interfaces. These types of optical interfaces are LC, SC, MPO, and CS. And there are also electrical copper transceiver modules using the RJ-45 interface. Among these interfaces, the LC interface can be divided into duplex and simplex, and there are dual-simplex LC interface (such as CSFP). For BiDi optical transceivers, there are also simplex SC interface, in addition to the simplex LC. We will introduce each of these interfaces one by one, according to the transmission modes of the transceiver modules.
LC/SC
As we konw, a transceiver module consist of a transmiter and a receiver. This means that the transmission has two directions. For the common single-channel optical transceivers, such as SFP28, SFP+, and SFP, the transmitting terminal is connected to one optical fiber and the receiving terminal is also connected to one optical fiber. That's why the common optical transceivers are called dual-fiber transceiver generally. The dual-fiber transceiver has a duplex LC interface connected to a duplex LC patch cable. (The XENPAK, X2, and GBIC dual-fiber transceivers, not listed in the table, have a duplex SC interface connected to a duplex SC patch cable.)
The single-fiber bidirectional transmission mode is called BiDi for short. The BiDi signals in both directions are combined in a single fiber. The bidirectional transmission means that the light is directional and will not interference each other. The BiDi optical transceiver, such as BiDi SFP+ and BiDi SFP, have a simplex LC or SC interface connected to a simplex LC or SC patch cable. And for high-density BiDi transmission networks, there are 2-channel BiDi SFP+/SFP (CSFP+/CSFP) optical transceivers using dual simplex LC interface.
MPO
For multi-channel optical transceivers, such as 4-channel QSFP+, 4-channel QSFP28, and 8-channel QSFP-DD, there are several Tx and several Rx. Some of them (such as 100G QSFP28 SR4 and 100G QSFP28 PSM4) have MPO interfaces, that is, multi-fiber pull on/off, using multiple optical fibers for the parallel transmission shown as the figure below.
There are also dual-fiber 4-channel optical transceivers using the multiplexing transmission mode, that is, multiple Tx multiplexing and Rx demultiplexing. These optical transceivers, such as 40G QSFP+ LR4 and 100G QSFP28 CWDM4, use two optical fibers for long-distance transmission, saving more optical fiber resources than using multi-core optical fibers. Like the common single-channel optical transceiver, the dual-fiber 4-channel optical transceiver also has a duplex LC interface connected to a duplex LC patch cable.
CS
The QSFP-DD MSA specification defines an 8-channel module, cage and connector system. The cage and connector system provides backward compatibility to the 4-channel QSFP28 modules. Doubling the number of duplex optical links with the QSFP-DD specification requires a new smaller optical interconnect to fit in the same physical cage form factor. For the eight-channel QSFP-DD optical transceivers using the multiplexing transmission mode, a new type of optical interface called dual CS is used to replace the duplex LC. The dual CS interface is connected to the CS connector, a miniature single-position plug which is characterized by duo cylindrical, springloaded butting ferrule(s) of a 1.25 mm typical diameter, and a push-pull coupling mechanism. The CS connector provides the characteristics and simplicity of the duplex LC connector into a smaller footprint to allow 2 pairs of CS connectors to fit within the physical constraints of the QSFP-DD form factor.
RJ-45
The RJ-45 interface is used in copper transceiver modules, such as 10G copper SFP+, 1G copper SFP and 100M copper SFP. The copper SFP+ transceivers transmit electrical signals over Category 6a or Category 7 copper cables with RJ-45 connectors, while the copper SFP transceivers transmit electrical signals over Category 5 or Category 5e copper cables with RJ-45 connectors.
Article Source: http://www.gigalight.com/news_detail/newsId=430.html
The Trend of DSP’s Application in Data Center
The data center 100G has begun to be used on a scale, and the next-generation 400G is expected to begin commercial use by 2020. For 400G applications, the biggest difference is the introduction of a new modulation format, PAM-4, to achieve a doubled transmission rate at the same baud rate (device bandwidth). For example, the single-lane baud rate of DR4 used for transmissions up to 500m need to reach 100Gb/s. In order to realize the application for such rate, the data center optical transceiver modules began to introduce Digital Signal Processor (DSP) chips based on digital signal processing to replace the clock recovery chips of the past to solve the sensitivity problem caused by insufficient bandwidth of the optical devices. Can DSP become a broad solution for future data center applications as expected in the industry? To answer this question, it is necessary to understand what problems the DSP can solve, what its architecture is, and how the development of its costs and power consumption trends in the future.
The Problems that DSP Can Solve
In the field of physical layer transmission, DSP was first applied in wireless communications for three reasons. First, the wireless spectrum is a scarce resource, and the transmission rate demand has been increasing. Increasing the spectrum efficiency is a fundamental requirement for wireless communications, so DSP is required to support a variety of complex and efficient modulation methods. Second, the transmission equation of the wireless channel is very complicated. The multipath effect, and the Doppler effect in the high-speed motion, can't satisfy the wireless channel's compensation demand with the traditional analog compensation. DSP can use various mathematical models to compensate the channel well Transmission equation. Third, the Signal-to-Noise Ratio (SNR) of the wireless channel is generally low, and the Forward Error Correction (FEC) should be used to improve the sensitivity of the receiver.
In the field of optical communications, DSP was first commercially used in long-distance coherent transmission systems over 100G. The reason is similar to that of wireless communications. In long-distance transmission, since the laying cost of optical fiber resources is very high, the improvement of spectral efficiency to achieve higher transmission rates on a single optical fiber is an inevitable requirement for operators. Therefore, after the use of WDM technology, the use of coherent technology based-on DSP has become an inevitable choice. Secondly, in long-distance coherent transmission systems, by using of a DSP chip, the dispersion effects, non-linear effects caused by transmitter (Tx) and receiver (Rx) devices and the optical fiber itself, and phase noise introduced by the Tx and Rx devices, can be easily compensated without the need for Dispersion Compensation Fiber (DCF) that placed in the optical link in the past. Finally, in long-distance transmission, due to the attenuation effect of optical fibers, an optical amplifier (EDFA) is generally used to amplify the signal every 80km to reach a transmission distance up to 1000km. Each amplification will introduce noise to the signal, reducing the SNR of the signal, therefore, the FEC should be introduced to improve the receiver's receiving ability during long-distance transmission.
To sum up, DSP can solve three problems. First, it supports high-order modulation formats and can improve the spectral efficiency. Second, it can solve the effects caused by components and signal-channel transmission. Third, it can solve the SNR problem.
Then, whether there are similar requirements in data center has become an important basis for us to judge whether we should introduce DSP.
First of all, let's take a look at the spectrum efficiency. Does data center need to improve spectrum efficiency? The answer is yes. But unlike the lack of wireless spectrum resources and insufficient optical fiber resources in the transmission network, the reason for improving spectrum efficiency in data center is that the insufficient bandwidth of the electrical/optical devices and the insufficient number of wavelength division/parallel paths (limited by the size of optical transceiver modules). Therefore, to meet the needs of future 400G applications, we must rely on increasing the single-lane baud rate.
The second point is that for single-lane 100G and above applications, current Tx electrical driven chips and optical devices can not reach bandwidths above 50GHz. Therefore, it is equivalent to that a low-pass filter is introduced at the transmitter. The performance on the code is inter-symbol interference in the time domain. Taking the application of 100G PAM-4 as an example, the bandwidth-limited modulation device will make the width of the optical eye diagram of the signal very small, then the clock recovery based on the analog PLL in the past could not find the best sampling point, making the receiver unable to recover the signal (this is also why the TDECQ needs to introduce an adaptive filter for equalization in the standards). After introducing the DSP, the signal can be directly spectrally compressed at the Tx end. For example, the extreme approach is to artificially introduce intersymbol interference between two symbols to reduce the signal bandwidth of the Tx end. At this time, the eye diagram of PAM-4 on the oscilloscope will become PAM-7 form. The Rx end recovers the signal through an adaptive FIR filter. In this way, the uncontrollable analog bandwidth effect in the modulating/receiving device becomes a known digital spectrum compression, reducing the bandwidth requirement for the optical device. Fujitsu's DMT (Discrect-Multi-Tone) modulation technology, which has been promoted in conjunction with DSP, can even use a 10G optical device to transmit 100G signals.
Third, does FEC technology really need to be introduced at the module end? Inside the data center, the maximum transmission distance is not more than 10km. The link budget is about 4dB with the loss of the joint. Such SNR effects caused by the link is basically negligible. Therefore, the FEC in the data center is not intended to solve the link SNR, but to solve the performance shortage of the optical devices. At the same time, we need to consider that the electrical interface signal at the optical module end is upgraded from 25G NRZ to 50G PAM-4 (net rate) in the 400G era, so it is often necessary to turn on the electrical FEC to meet the requirements for transmission from the optical transceivers to the switches. In this case, reopening the FEC on the module side is not necessary and has no effect. Because for FEC, we mostly discuss the error correction threshold. For example, 7% FEC error correction threshold is at 1E-3 Bit Error Rate (BER), that is to say that FEC is able to correct all errors below this BER, and FEC above this BER is essentially useless (regardless of the Burst Error which is usually solved with Inter-leaver). Therefore, there is no difference between the effect of using multiple FECs and using only the best FEC. Considering the power consumption and delay caused by FEC on the module side, it may be better to open FEC on the switch side in the future.
The Architecture of DSP
In the optical communication field, DSP generally consists of several parts: the front-end analog digital mixing section, including ADC (Digital-to-Analog Converter, required), DAC (Analog to Digital Converter, optional) and SerDes, digital signal processing section (including FEC) ) and the PHY section. The PHY section is similar to the CDR chip with the PHY function, and will not be described here.
The main function of ADC and DAC is to convert analog signal and digital signal, which is a bridge between the modulation device and digital signal processing section. The ADC/DAC mainly has four key indicators which are sampling rate, sampling effective bit width, analog bandwidth and power consumption. For the 100G PAM-4 application, the sampling rate of ADC in the Rx end needs to reach 100Gs/s. Otherwise, Alias will be generated during sampling, which will cause distortion to the signal. The effective sampling width is also very important. For PAM-4 applications, it does not mean that 2 effective bits can satisfy the requirement of digital signal processing, but at least 4. Analog bandwidth is currently the main technical challenge for ADC/DAC. This index is limited by both effective bit widths and power consumption. Generally, there are two ways to implement high bandwidth ADC/DAC which are GeSi and CMOS. The former has a high cutoff frequency and can easily realize the high bandwidth. The disadvantage is very high power consumption, so it is generally used in instrumentation. The cutoff frequency of CMOS is very low, so to achieve high bandwidth, multiple sub-ADCs/DACs must be sampled using an interleaving method. The advantage is low power consumption. For example, in a coherent 100G communication system, a 65Gs/s ADC with 6 effective bits is composed of 256 sub-ADCs with a sampling rate of 254Ms/s. It must be noted that although the ADC has a sampling rate of 65Gs/s, its analog bandwidth is only 18GHz. With a clock jitter of 100fs, the theoretical maximum analog bandwidth of 4 effective bits width is only up to 30GHz. Therefore, an important conclusion is that under the condition of using DSP, the bandwidth limitation of the general system is no longer the optical device, but the ADC and DAC.
In the data center applications, and the digital signal processing unit is still relatively simple. For example, for 100G PAM-4 applications, it performs spectral compression of the transmitted signal, nonlinear compensation, and FEC encoding (optional) in the Tx end, then the ADC uses an adaptive filter to compensate the signal and digital domain CDR in the Rx end (separate external crystal support is required). In the digital signal processing unit, the FIR filter is generally used to compensate the signal. The Tap number and the decision function design of the FIR filter directly determines the performance of the compensation DSP and power consumption. It should be particularly pointed out that the DSP application in the field of optical communications is facing with a large number of parallel computing problems. The main reason is the huge difference between the ADC sampling frequency (tens or even 100Gs/s) and the digital circuit operating frequency (up to several hundred MHz), in order to support the ADC of 100Gs/s sampling rate, digital circuits need to convert the serial 100Gs/s signals into hundreds of parallel digital signals for processing. It can be imagined that when the FIR filter only adds one Tap, the actual situation is that hundreds of Taps needs to be added. Therefore, how to deal with the balance of performance and power consumption in the digital signal processing unit is the key factor to determine the quality of the DSP design. In addition, inside the data center, optical transceiver modules must meet the interoperability prerequisites. In practical applications, the transmission performance of a link depends on the overall performance of the DSP and analog optical devices in the Tx and Rx ends. It is also a difficulty to design a reasonable standard to correctly evaluate the performance of the Tx and Rx ends. When the DSP supports that FEC function is opened in the the physical layer, how to synchronously transmit and receive the FEC function of the optical transceivers also increases the difficulty of data center testing. Therefore, so far, coherent transmission systems are interoperable among manufacturers' devices, and do not require interoperability among different manufacturers. (The TDECQ performance evaluation method is proposed for PAM-4 in 802.3.)
Power Consumption and Cost
Because DSP introduces DAC/ADC and algorithm, its power consumption must be higher than the traditional CDR chip based on simulation technology. And the method that DSP lowers the power consumption is relatively limited, mainly depending on the promotion of the process of tape, for instance, upgrading from the current 16nm to 7nm process can achieve a 65% reduction in power consumption. The current design power consumption of the 400G OSFP/QSFP-DD based on the 16nm DSP solution is around 12W, which is a huge challenge for the thermal design of the module itself or the future front panel of the switch. Therefore, it may be based on the 7nm process to solve the 400G DSP problem.
Price is always a topic of concern to data center. Unlike traditional optical devices, DSP chips are based on mature semiconductor technology. Therefore, larger chip costs can be expected to fall under the support of massive applications. Another advantage of DSP's future application in data centers is flexibility, which can meet the application requirements of different data rates and scenarios by adjusting the DSP configuration in the same optical device configuration.
Article Source: http://www.gigalight.com/news_detail/newsId=422.html
Related Gigalight 100G QSFP28 Optical Transceivers:
Gigalight Launches Industrial-Grade 100G QSFP28 Optical Transcei
Shenzhen, China, April 30, 2018 − Recently, Gigalight has successfully developed two industrial-grade 100G optical transceivers: 100G QSFP28 LR4 (up to 10km) and 100G QSFP28 4WDM-40 (up to 40km). At the same time, these new products have been implemented in a small batch of trial production.
The Gigalight industrial-grade 100G QSFP28 LR4 transceiver is designed with the reliable Japanese industrial-grade TOSA in the transmitting end, and adopts our high-quality self-developed thermal-design ROSA in the receiving end. In the condition of full temperature of -40 to 85 degrees, there is an excellent performance with a margin more than 31% in the Eye Diagram (as shown in the figure below) . Moreover, it has a power consumption less than 3.5W and can meet the demands of 10km fiber transmission with zero-error-rate at 100GE data rates. It is fully compliant with IEEE 802.3ba 100GBASE-LR4 standard and is an ideal choice for applications of relatively harsh environments, meeting customers' demands of particular applications and the optical transport network transmission between AAU and DU for the future 5G mobile fronthaul application.
Gigalight Industrial-grade 100G QSFP28 LR4
Eye Diagram of Gigalight Industrial-grade 100G QSFP28 LR4 under -40℃ (left) and -85℃ (right)
The Gigalight industrial-grade 100G QSFP28 4WDM-40 transceiver adopts the reliable Janpanese industrial-grade TOSA and ROSA on both the transmitting and receiving ends. It has a low power consumption less than 3.8W at the full temperature range of -40 to 85 degrees, ideal for building green data center and reducing energy costs. Its ROSA adopts a highly sensitive APD photodetector with a sensitivity less than -16.5dBm. When the FEC function is enabled in the system side, it can transmit up to 40km and meet the requirements of the 100GE 4WDM-40 MSA specification. This optical transceiver provides a cost-effective long-distance Data Center Interconnection (DCI) solution for distributed data centers under harsh environments, and also meets the demends of the optical transport network transmission between AAU and DU for the future 5G mobile fronthaul application.
Gigalight Industrial-grade 100G QSFP28 4WDM-40
5G Fronthaul Network
There is no doubt that the future 5G network will more rely on the support of optical network. Meanwhile, the fronthaul to 5G needs a strong optical network due to the flat network architecture. Besides, the density and increasing number of base stations will lead a tremendous demand for optical fiber resources and broadband. The successful launch of industrial-grade 100G QSFP28 optical transceivers has not only enriched the 100G QSFP28 product line but also created a product line with unique features, which can better serve the 5G optical transmission demands and supply the gap in the market.
About Gigalight:
Gigalight is global optical interconnection design innovator. A series of optical interconnect products include: optical transceivers, passive optical components, active optical cables, GIGAC MTP/MPO cablings, and cloud programmers & checkers, etc. Three applications are mainly covered: Data Center & Cloud Computing, MAN & Broadcast Video, and Mobile Network & 5G Optical Transmission. Gigalight takes advantage of its exclusive design to provide clients with one-stop optical network devices and cost-effective products.
A Comparison between CWDM and DWDM
Transporting a single data stream through an optical fiber can be costly and inefficient. Wavelength Division Multiplexing (WDM) can transmit multiple, independent data streams through a single optical fiber to better utilize the bandwidth of the given optical fiber. Each data stream adopts a different wavelength of light (also know as lambda λ) and the data streams travel through a single fiber. With WDM, multiple cloud IaaS tenants can share the same optical fiber. Closely spaced wavelengths provide a higher number of channels (or bandwidth) per fiber. According to the wavelength spacing, WDM technology can be divided into two parts: Coarse WDM (CWDM) and Dense WDM (DWDM).
For many SAN engineers, DWDM is the ideal SAN extension mechanism since it provides very high scalability, very low and predictable latency, and moderately long distances. With regeneration, the point-to-point distance for a DWDM link can be extended further. However, additional latency is incurred during the regeneration process. The maximun distance for a DWDM link is dependent on the components used along the link, such as optical amplifiers, DCUs, VOAs, OADMs, and so on.
Tips: As a rule of thumb, the maximum point-to-point distance is about 200 km with amplification only (i.e., no regeneration).
CWDM, just like DWDM, uses multiple light wavelengths to transmit signals over a single optical fiber. Nevertheless, the two technologies vary in many ways:
1. CWDM uses a 20-nm wavelength spacing that is much wider than the 0.4-nm for DWDM. The wider wavelength spacing in CWDM means lower product development costs. This is one reason why CWDM is less costly than DWDM.
Note: Most CWDM devices (i.e., CWDM MUX/DeMUX) operate in the 1470-nm to 1610-nm range. The frequency grid for DWDM and the wavelength grid for CWDM systems are defined by the International Telecommnications Union (ITU) standards G.694.1 and G.694.2, respectively.
- 2. CWDM provides a maximun of 8 lambdas between two CWDM multiplexers over a single fiber pair as compared to DWDM, which support up to 32 lambdas (based on 0.8-nm or 100-GHz wavelength spacing) over a single fiber pair.
Note: Some long-haul DWDM sytems can support up to 160 lambdas per fiber pair.
3. Each CWDM channel uses a specialized GigaBit Interface Converter (GBIC) or small form-factor pluggable (SFP) transceivers. These specialized transceivers are commonly known as colored GBIC and SFP. Each CWDM channel uses a different "color" GBIC or SFP because each lambda represents a different color in the spectrum. In this case, the native GBIC or SFP in the client devices are substituted with a colored GBIC or SFP. You can buy the most cost-effecitve CWDM SFP from Fiberstore.
- 4. CWDM multiplexers are usually passive (i.e., not powered) devices containing a very accurate prism to multiplex eight separate wavelengths of light along a single fiber pair. Passive devices can't generate or repeat optical signals.
- 5. No amplification is possible with CWDM because CWDM uses wavelengths that can't be amplified with EDFA amplifiers. Therefore, the maximum distance for a CWDM link is approximately 100 km.
Note: The Cisco ONS 15501 EDFA, which has a wavelength range of 1530 nm to 1563 nm. can only amplify two signals (1530 nm and 1550 nm) out of the eight signals that are multiplexed onto the fiber pair.
- 6. CWDM provides an alternative solution to DWDM for low-latency and high-bandwidth requirements associated with synchronous replication applications. However, DWDM is more scalable than CWDM. DWDM also has longer diatance capacity than CWDM because DWDM can be amplified (using a DWDM EDFA). The main benefit of CWDM is its low cost. It is a cheaper solution than DWDM. In other words, CWDM is optimized for cost, while DWDM is optimized for bandwidth. For enterprises that have access to dark fiber and have only limited scalability requirements, CWDM is a relatively inexpensive way to achieve low-latency and high-bandwidth interconnections between DCs. The CWDM implementation also results in less complex installation, configuration, and operation as compared to DWDM.
- 7. CWDM can also be used to enable multiple ISL connections between the switched over a single fiber since it requires less fiber for interconnecting two metro sites. The same benefit applies to port channel implementation between the switched.
In short, DWDM is a solution that provides a higher number of connections and longer reach, or extension, at a much higher cost which CWDM is a more cost-effective solution for metro or campus solutions where the distance is limited.
Article source: http://www.fiberopticshare.com/
RoHS Compliant NETGEAR AGM732F for Gigabit Ethernet and Fibre Channel Applications
NETGEAR AGM732F is a RoHS compliant SFP transceiver module designed expressly for high-speed communication applications that require rates of up to 1.25 Gbps. It is compliant with the Gigabit Ethernet standards, as well as the SFP Multi-Source Agreement (MSA).
The AGM732F module provides with the LC receptacle that is compatible with the industry standard LC connector. It is also compatible with industry standard RFT connector and cage. The post-amplifier of the AGM732F includes a LOS (Loss Of Signal) circuit that provides a TTL logic-high output when an unusable optical signal level is detected. AGM732F is a Class 1 eye safety product. Its optical power levels are at eye safe level under normal operation.
NETGEAR AGM732F MODULE'S FEATURES
- Compliant with SFP transceiver MSA specification
- Compliant with specifications for IEEE 802.3z/Gigabit Ethernet
- Compliant with the 1.0625GBd Fibre Channel 100-SM-LC-L FC-PI Rev.13
- Compliant with industry standard RFT Electrical connector and cage
- Single + 3.3 V power supply and TTL logic interface
- EEPROM with serial ID functionality
- Laser Class 1 product which complies with the requirements of IEC 60825-1 and IEC 60825-2
- Duplex LC connector interface
NETGEAR AGM732F MODULE'S APPLICATIONS
- Gigabit Ethernet
- Fibre Channel
- Switch to Switch interface
- Switched backplane applications
- File server interface
NETGEAR AGM732F MODULE'S PERFORMANCE
- Data Link up to 10 km in 9/125 µm Single Mode Fiber (SMF)
NETGEAR AGM732F MODULE'S PARAMETERS
NETGEAR AGM732F MODULE'S DIMENSION
Fiberstore NETGEAR AGM732F SFP'S (compatible) SPECIFICATIONS
- Manufacturer: Fiberstore (the label can be customized)
- Product Type: SFP transceiver module
- Data Rate: 1 Gbps
- Wavelength: 1310 nm
- Transfer Distance: 10 km
- Connector Type: LC Duplex
- Cable Type: Single-Mode Fiber (SMF)
About Fiberstore:
Fiberstore designs, develops, manufactures and markets a diverse portfolio of high-performance, cost- competitive products for all fiber optic communication applications. The compamy supplies a series of compatible NETGEAR SFP modules (NETGEAR AGM731F, AGM734, etc.), i.e. SFP-1G31-10-NE as a compatible version of NETGEAR AGM732F, according to customers' requirements. They also design a customized label and special packaging for users' companies. Their primary corporate capabilities include software engineering, systems engineering, network engineering, systems support, program management, and information assurance engineering and analysis.
Article source: http://www.fiberstore.com/blog/rohs-compliant-netgear-agm732f-for-gigabit-ethernet-and-fibre-channel-applications.html
The Rapid Development of Optical Transceiver Market
Several years ago, there was a report which said that the 10G/40G/100G optical transceivers would hit 1.44 billion dollars by 2014, driven by 10G SFP+ and tunable XFP modules. It is now 2014, and the forecasts have came true.
Infontetics Research released the report tracking 10-Gigabit (10G), 40-Gigabit (40G), and 100-Gigabit (100G) optical transceivers and transponders sold into the optical transport, carrier routing and switching, and enterprise markets.
In fact, the highlights of optical transceiver market have appeared years ago. Let's make a review.
- 10G, 40G, and 100G transceiver and transponder revenue was forecast by Infonetics Research to grow to 1.44 billion dollars worldwide by 2014, driven by SFP+ and tunable XFP technology, and by spikes in future 40G and 100G adoption.
- Tunable XFPs would be the major 10G growth opportunity for a long time, as they eliminated inventory management issues with fixed-wavelength modules, fulfilled the need for tunability as ROADM-based networking rises in popularity, and replaced the more-costly 300-PIN format.
- Meanwhile, SFP+ would replace XFP in the future, driven by strong growth in 850nm modules for 10-Gigabit Ethernet (10GbE) and 8/16G Fibre Channel (FC) applications.
- Shipments of next-generation 40G long-range (LR) and short-range (SR) optical transceivers would begin in 2010, primarily for enterprise and IP router applications.
Revenue for 40G long-reach interfaces jumped 52 percent in 2009 over 2008, to 114.6 million dollars, while revenue for 40G 300-PIN short- and intermediate-reach interfaces dropped 35 percent, driven by price erosion and demand weakness.
- During the 2011-2012 timeframe, shipments of DQPSK transceivers were expected to outstrip those of DPSK, as the cost differential between the two drops. Worldwide revenue was forecast to grow nearly 10-fold from 2009 to 2014 for the tunable DQPSK 40G fiber transceiver.
Infonetics' report provided in-depth analysis, market size, and forecasts through 2014 frp manufacturer revenue and units shipped for 10G, 40G, and 100G transceivers and transponders. Specifically, the report tracked the following long- and short/intermediate-reach optical transceivers/transponders.
- 10G modules by tunable, WDM (fixed C-band), 1550nm,1310nm, and 850nm wavelengths, split by form-factor such as 300-PIN, SFP+, XFP, X2, XENPAK etc..
- 40G modules by tunable, 1310nm and 850nm wavelengths, split by form-factor such as DPSK, DQPSK, opto duo-binary (ODB) and other, 300-PIN SFI-5, 40GBASE-LR4, and 40GBSE-SR4.
- 100G modules by tunable, 1310nm and 850nm wavelengths, split by form-factor such as 100G DWDM, 100GBASE-LR4 (aka 4*25G non-return-to-zero, or NRZ), and 100GBASE-SR10 (aka 10*10G).
Nowadays, vendors providing products in the optical transceiver market include Cisco, Finisar, Avago, JDSU, and so on. Besides these famous companies, there are also many other manufacturers and suppliers who provide compatible transceivers with much more cheaper prices. For example, the compatible Finisar 10G SFP+ modules in FiberStore are all under 100 dollars!
PON Transceiver Modules for FTTH
Optical transceivers are classified by wavelengths, data rates, reaches, packaging types, electrical and optical interfaces, temperature ranges, etc. The mannfacturing followings a process from discrete optics to optical subassembly and integrated chips to module assembly and test. There are various optical transceivers currently used with optical access systems. Conventional transceiver technologies based on discrete optoelectronic chips, components, and coaxial packaging are still the key enabler for the industury. The high cost of transceivers is one of the major barriers to mass deployment.
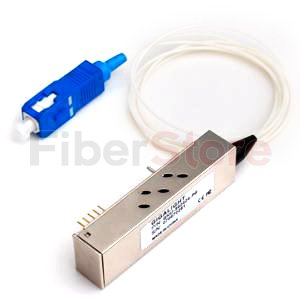
PON transceivers are bidirectional devices that use different wavelengths to transmit and receive signals between an OLT at a central office (CO) and the ONTs at end users' premises. There are currently two standard types of transceivers: the diplexer and the triplexer transceivers, respectively. For the diplexer transceivers, the wavelengths are designed in accordance with industry standards, namely, 1310 nm for the upstream and 1490 nm for the downstream wavelengths. For triplexer transceivers, the 1550 nm wavelength is allocated for analog broadcast video overlay in the downstream direction. It is also possible that digital video signals are carried on the downstream 1490 nm wavelength using video over IP technologies.
For rapid adoption of FTTH, it is important to reduce the cost of optical transceivers. In particular, the overall PON system cost is weigthed more toward the ONU, as the OLT cost is shared among the number of FTTH users. So the ONU transceiver should be the main target for cost reduction whenever possible. In summary, the technological challenges in the optical transceivers for PON systems exist in the following areas:
1. High-output-optical-power and high-sensitivity OLT at the CO to compensate for the losses introduced by the optical splitter and the transmission fibers connecting subscribers' premises.
2. Burst-mode optical transmission technologies for the upstream link.
3. Cost-effective packaging of optical devices.
4. Integration of more digital and analog functions into a single IC.
PON transceivers still represent a very active area in industry research and development due to the huge market opportunity. Because of the PON point-to-multipoint nature, PON burst-mode transceiver must face several unique characteristics to meet the requirements. Despite widespread interest, there is still no related PON transceiver multisource agreement (MSA) defined to support this application. Optical module vendors normally collaborate with system vendors on a case-by-case basis in specifications for diverse systems. Because of the EPON influence, SFF and SFP MSAs gained some popularity, while other form factors like GBIC and proprietary triplexer designs still exist for this application.
From the EPON/G-PON system compatibility and reusability viewpoint, transceiver modules can be split into blocks of BOSA, electrical subassembly (ESA), housing, and thermal management. The BOSA module must satisfy transmitter optical power, and receiver sensitivity, etc. Considerations on the ESA side include burst-mode physical-media-dependant (PMD) drving performances, system control signal acceptable, high receiver sensitivity, and receiver circuits also need to be examined. One most important aspect is the specific testing items such as transmitter ON/OFF time and receiver settling time. It is of key importance for system designers to maintain system performance and utilize system data processing ability. On the mechanical side, many critical issues need to be considered, such as thermak, EMI, temperature, humidity effect, etc.
Besides the PON transceivers, the biggest Chinese transceiver modules manufacturer FiberStore also provides SFP port to RJ45 Netgear and Netgear SFP+ module such as 10GbASE-LR SFP+ module Netgear.